New MRI Technology Sheds Imperfections
Magnetic resonance imaging (MRI) uses a large magnet and radio waves to noninvasively examine organs, soft tissues, bone, and virtually all other internal body structures. It has been used since the 1970s to diagnose and treat medical conditions such as brain tumors, internal bleeding, and torn ligaments.
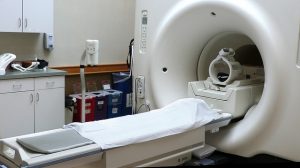
This traditional MRI machine can create images with imperfections. Researchers are hoping this new technology will revolutionize MRIs. (Flickr / Creative Commons / Liz West)
Although much has been invested in magnetic coils to force uniform imaging, many MRIs still yield dark artifacts. A new technology harnesses the dark artifacts to create images resolved enough to create consistent diagnosis across populations for the first time. The study was led by NYU’s Langone Medical Center and published August 16th in the journal Nature Communications.
MRIs have become an unrivaled diagnostic technique able to map tissue anatomy and physiology. The technology is part of virtually every area of medicine, with U.S. physicians ordering upwards of 28 million per year.
Despite this, residual radiofrequency (RF) field inhomogeneities appear, leading to artifacts that compromise the diagnostic and scientific values of the images.
While someone undergoes a scan, antennas hit the atoms making up tissues with radio waves that knock them out of their magnetic equilibrium. When this happens, the atoms’ magnetic forces spin to reveal their identity and position. But the quality of the image depends on the equal exposure of atoms to magnetic fields. These vary from machine to machine, and in their interactions with different parts of even a single tissue sample.
So, the darkened artifacts are basically unavoidable with the current system. The traditional MRI lights up some areas better than others, with imperfections blacking out some areas. The industry has even invested in magnetic coils to force uniform imaging, but, again, the imperfections are simply unavoidable.
The study author was Daniel Sodickson, MD, PhD, and professor in the Department of Radiology. He is also a member of the Perlmutter Cancer Center at NYU Langone and director of the Bernard and Irene Schwartz Center for Biomedical Imaging. He said that the advances in this new study eliminates artifacts and creates images accurate enough to assign numerical values to anatomical features.
“This marks the advent of ‘quantitative MRI,’ where new kinds of tissue maps become the gold standard for diagnoses and reveal disease patterns that are consistent from patient to patient,” says Sodickson, also lead investigator for the Center for Advanced Imaging Innovation and Research. “It will turn scanner design on its head.”
The approach promises to accomplish superior imaging with less expensive machines. Faster, simpler approaches also eliminate the long time it takes to calibrate machines, which cause the MRI to last longer than the average patient can tolerate.
Even still, traditional MRI approaches yield only qualitative images not resolved enough to generate data-driven diagnoses.
To overcome these challenges, Mark Griswold at Case Western Reserve University led work published in 2013, which described magnetic resonance fingerprinting (MRF). Before his work, MRI scanners had to wait for magnetic spins to return to their normal equilibrium between each sequential radio wave pulse, hindering speedy imaging. MRF technology built images based on complex interplays of overlapping signals.
MRF images were built on a small amount of information from the scanner matched to databases of known tissue patterns. The method replaced slower methods that required building the image from scratch. The first author of the current study, Martijn Cloos, PhD, NYU Langone magnetic resonance (MR) physicist and an assistant professor in the Department of Radiology, saw that MRF technology was still lacking. It was held back by its attempt to match real spins to patterns of a simulated scanner calibrated to offer perfectly uniform exposure.
Still, MRF did not offer perfect images without artifacts. To correct this, Cloos and colleagues created “Plug-and-Play MR Fingerprinting” (PnP-MRF), throwing out the idea of the ideal scanner.
Artifacts can be eliminated by deliberately interweaving freely varying heterogeneous radio frequency fields into a magnetic resonance fingerprinting data-acquisition process. In other words, it matches its measurements to a simulated database of every possible magnetic field interaction or distortion as it builds images, requiring zero calibration. The new method captures spin characteristics while also effectively mapping distortions that occur as magnetic resonance radio waves interact with tissue. Radiologists previously sought to erase this using calibration. The authors said that these same field distortions can serve as a new set of tissue parameters that aid in diagnoses.
MRF used a single source of radio wave pulses to generate signals. This new technology is a circling strobe light of many broadcast magnetic fields, hitting the atoms from different directions separated by milliseconds to create a new kind of fingerprint. An artifact from any one radio wave pulse will not show up in all of the datasets, creating a dismissal of errors.
The new technology liberates the MRI from the traditional struggle for control over radio frequency uniformity.
The innovations enable PnP-MRF to correct for each scanner’s peculiarities and magnetic field-tissue interactions, which were confirmed by a series of numerical and tissue experiments.
The design has allowed the complexity in creating MRI images to move from machinery to computation, Cloos said.
“Rather than building chambers to house extensive magnet coils that fight non-uniformities, near-future scanners, by embracing heterogeneous fields, will consist of simple tabletop magnets, or possibly even hand-held MRI wands,” he added.